The neocortex is an evolutionarily new part of the brain unique to mammals and is responsible for high level sensation, movement and cognition. It wouldn't be fair to summarize in a sentence or two what cortex "does," but it is clear that it is an important part of the brain. Korbinian Brodmann famously divided the human cortex into about 50 areas based on histology of the six cortical layers in different parts of the brain; Brodmann's areas are still used today because their functions follow their histological structure. While cortical areas have largely stereotyped wiring patterns, some connectivity “motifs” are thought to be area-specific, varying based on the type of input the area receives. It is unknown whether the type of input (i.e. statistics of incoming activity that vary with types of sensory stimuli) to a given cortical area determines the types of connectivity motifs present in that region. And while classical cortical “rewiring”experiments from Mriganka Sur’s lab have shown that primary sensory cortices are somewhat tolerant to process foreign inputs, it is not clear to what extent those circuits constitute basic computational units or if foreign inputs cause reorganization of connections within the circuit.
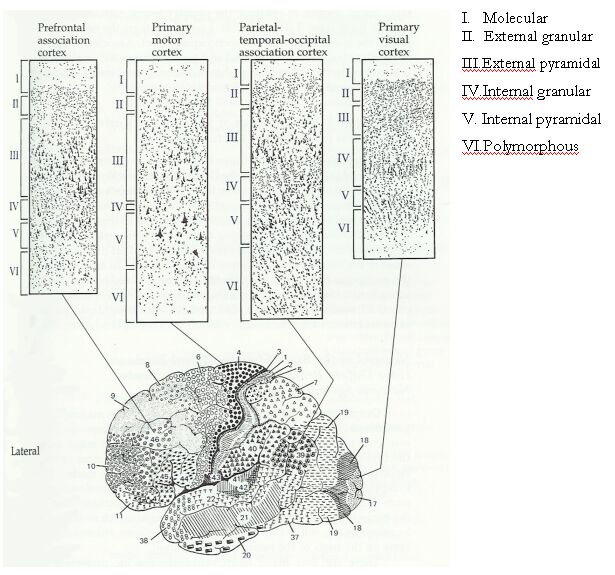
In a paper published in PLoS Biology this February, Dennis Katzel and Gero Miesenbock tested if inhibitory connections to pyramidal neurons in layer 2/3 within columns of mouse barrel cortex undergo plasticity following sensory deprivation (whisker cutting) and recovery (whisker regrowth). Using raster optogenetic ChR2 stimulation of batches of inhibitory neurons while recording post-synaptic responses in L2/3 pyramidal neurons in brain slices, they found that whisker trimming causes inhibitory input to L2/3 pyramidal cells from L5B to decrease fivefold and input from L1 to increase twofold; these connectivity changes reverse when the whisker regrows.
Furthermore, the changes in connectivity affect not only the deprived areas, but neighboring cortical columns that represent spared whiskers, even though deprivation does not change the horizontal extent of inhibitory connections. Finally, not only does the synaptic strength change after deprivation, but so does the total number inhibitory connections - half of L2/3 pyramidal neurons did not receive input from L5B interneurons (compared to 82% receiving input in control and recovered conditions). Together, these results suggest that cortical areas are able to undergo reorganization following changes in input statistics on an ongoing basis.
***
Science is inherently complex and nuanced, requiring years of schooling for one to properly understand its intricacies. As such, scientists have an obligation to present their work as clearly and simply as possible. Failure to do so prevents their ideas and hard work from spreading, resulting in a scenario where both scientist and reader (other scientists or the public) lose. As David Foster Wallace noted in his essay, “Authority and American Usage,”the author must always keep in mind what his/her reader knows and doesn’t know. Katzel and Miesenbock fail to keep the reader in mind: their paper is hard to read and almost impossible to understand.
For example, the paper does not explain the precise anatomical arrangement of the relevant whiskers; the reader is left assuming that whisker C sits linearly between whiskers B and D. Figure 3 leaves one wondering what a “standard barrel”is and how it is used to normalize the connectivity map (and why the “yellow outline”is put in layer 4?). From Fig 3C, it is unclear how it is possible that the unnormalized data reveals an additional statistically significant difference that does not show up in the normalized data.
The latter point makes the data seem unreliable, a sentiment which is also supported by the common occurrence throughout the paper of standard deviation values that are higher than the mean, which suggests the data is skewed by some outliers (which are evident in Figure 9). Furthermore, the authors claim that recovery from sensory deprivation (i.e. when the whiskers grow back) leads to recovery of the statistics of inhibitory connections to L2/3 pyramidal cells, but some of the data do not support this claim. For example, in their examination of the numbers of connections from L1, the authors write that the numbers of connections went “from 7.2 +/- 3.3 in control barrel cortex to 9.2 +/- 3.1 in deprived whisker columns, and returned to 5.5 +/- 3.1 and 8.5 +/- 3.5 during and after whisker regrowth.”Comparing the starting value (7.2 +/- 3.3) to the recovery value (5.5 +/- 3.1) reveals statistical differences (p = 0.0107, assuming normal distribution). By contrast, comparing the starting value to 9.2 +/- 3.1 in the deprived whisker reveals no significant difference (p = 0.89). It is misleading then to write that the original value “rose”and then “returned”to baseline.
One caveat of the data is that wide-field stimulation of ChR2 activates variable numbers of neurons. The authors use estimates from two previously published papers, which don’t agree on any given value (Table S1), to guess how many neurons their stimulation activates. The variability in the numbers of activated neurons could explain why the SD values tend to be high throughout the paper.
Overall, a paper should be judged by how well its data matches the claims it makes. The authors claim to answer the following questions with the data in this paper (questions are quoted from the paper):
- Is the presence of a specific wiring motif linked to the particular type of input a cortical area receives? In other words, does the motif change when the type of input changes?
- If so, is plasticity limited to a critical developmental period, or does the capacity to adapt persist into adulthood?
- And how motif-specific is the change?
- Are variable wiring motifs inserted or removed on demand, akin to plug-in devices that add new functionalities, or are circuits reconfigured more broadly?
The data show that 1) yes, the motifs change based on the type of input the area is receiving, but the manipulation of input is overly simplistic (standard input vs. no input at all). The question would be better answered by manipulating the type of input received, as in Sur’s rewiring experiments, or if the statistics of the input were altered to match another type of input.
An experiment to address this would be to raise animals in a controlled sensory environment where all acoustic stimulation comes through headphones that transmit a transform of the visual world to the headphones (“sensory substitution device”). This way, the original wiring is preserved, so the only manipulation is the statistics of the sensory input. Examining cortical wiring under this paradigm would be a more direct way of answering whether cortex is adaptable to the type of input it receives.
The data in this paper adequately addresses questions 2 and 3 - the plasticity seems to be intact in young adult animals, and the changes in wiring are motif-specific. Question 4 is rather open-ended and unclear, but one relevant result is intriguing - that the changes in connectivity following sensory deprivation of some whiskers (B and D) seem to affect spared ones (C) as well, not horizontally but in a column-specific way. Changes to connectivity in unaffected columns could imply that circuits configure “broadly,”on a scale larger than individual elements of sensory input (whiskers in this paradigm).